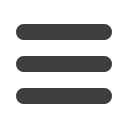
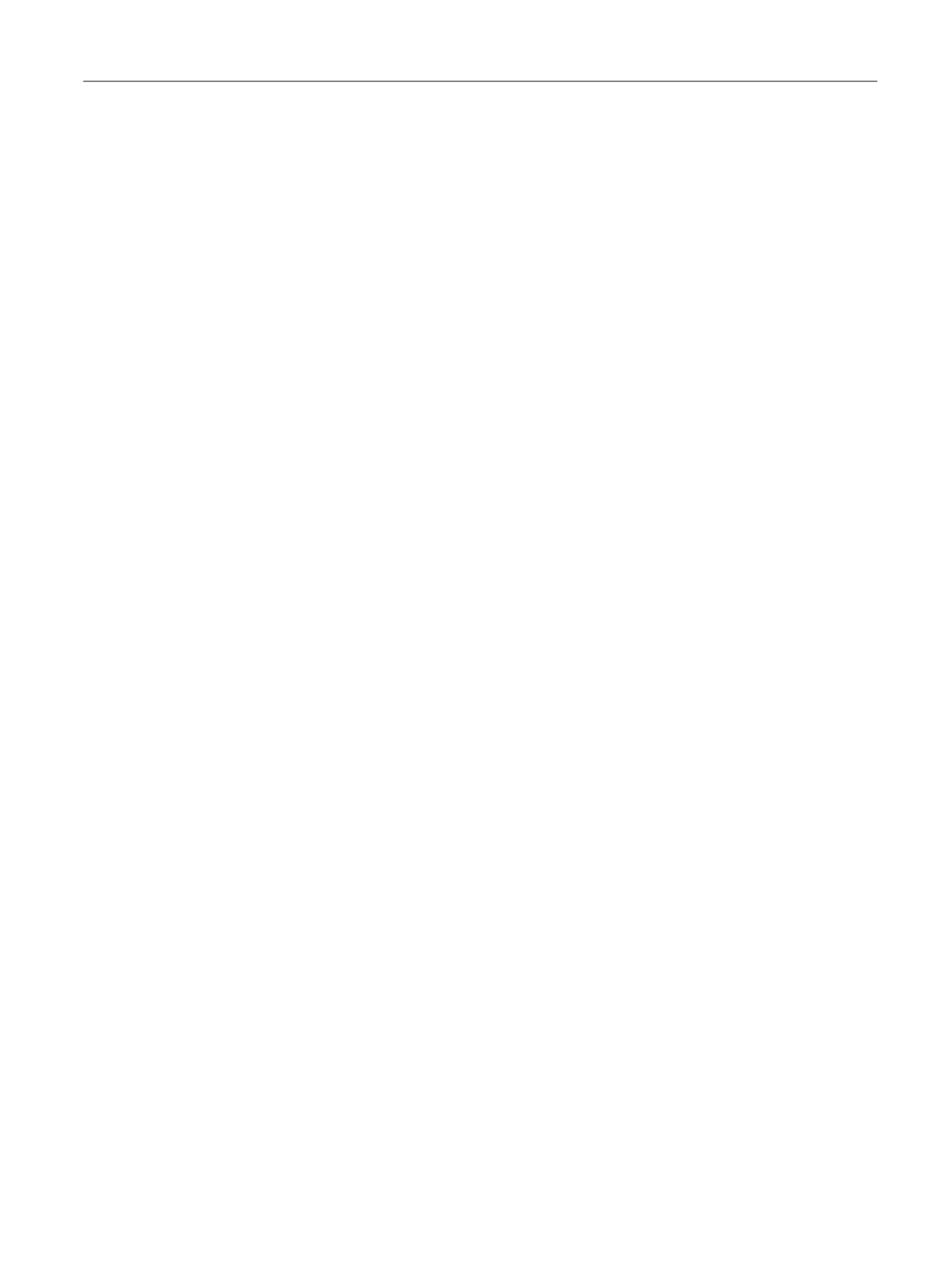
of intratumoral T cells in cancer have been described
[5]. First, the level of mutational load, and consequently the
presence of immunogenic neoantigens, may determine the
T-cell density
[6]. Second, trafficking towards and within
tumors and local activation of T cells may be hindered by
barriers that are raised by reactive vasculature, stroma, and
hypoxic areas
[7] ,which in turn may be linked to oncogenic
pathway disturbances in tumor cells
[8]. Bovinder Ylitalo
and colleagues now provide the first evidence that AR
signaling may be linked to exclusion of immune cells in
prostate cancer.
In general, prostate cancer is likely to be relatively
refractory to novel immune checkpoint blockade in
single-agent modality. The only immunotherapy that
has shown a survival advantage in prostate cancer so far
is sipuleucel-T. In a neoadjuvant study using sipuleucel-T,
infiltration of immune cells into the tumor periphery
increased. Moreover, broadening of the T-cell repertoire
could be detected in these tumors
[9,10]. However,
sipuleucel-T failed to lower prostate-specific antigen
levels or to yield objective clinical responses. With their
hypothesis, Bovinder Ylitalo and colleagues have pro-
posed a better interpretation of these data, as the increase
in the number of T cells may not be sufficient to attack
AR-dependent, MHC class I–deficient tumor cells. It is
interesting that Bovinder Ylitalo and colleagues found a
downregulated type I IFN pathway (IFNAR2), which may
explain the compromised immune profile in the high-AR
subgroup. This may in fact provide new options to treat
high-AR patients with drugs that stimulate such path-
ways and allow chemokine-driven recruitment of im-
mune effector cells
[8].
To guide future immunotherapy in prostate cancer we
need to further expand our knowledge of the T-cell infiltrate
in terms of numbers, locations, and subsets of T cells
[11]. More comprehensive studies should provide more
definite evidence as to whether the non–AR-driven
subgroup, as argued by the authors, may benefit from
checkpoint inhibitors to reactivate T cells already present in
prostate cancer. PD-L1 may be of particular interest, as its
expression is linked to the metabolic activity of tumor cells,
and could provide an additional avenue for treatment of the
non–AR-driven castration-resistant prostate cancer sub-
group. For the AR-driven subgroup, drugs that stimulate the
type I IFN response or immunomodulatory cytokines may
tip the balance towards sensitization of prostate cancer to
immunotherapies such as adoptive T-cell therapy and
checkpoint inhibitors.
In summary, Bovinder Ylitalo and colleagues present
provocative study results that may give rise to a novel
understanding of the role of immune cells in prostate cancer.
However, we do need to see independent confirmation
before these data can lead to more solid conclusions.
Immunotherapy studies in prostate cancer should include
basic research. Likewise, new studies should relate clinical
and translational findings to aberrations in the DNA repair
pathway; its major promise is the identification of a
subgroup of patients who respond better to current and
novel therapies.
Conflicts of interest:
The authors have nothing to disclose.
References
[1]
Mateo J, Carreira S, Sandhu S, et al. DNA-repair defects and olaparib in metastatic prostate cancer. N Engl J Med 2015;373:1697–708.
[2]
Watson PA, Arora VK, Sawyers CL. Emerging mechanisms of resis- tance to androgen receptor inhibitors in prostate cancer. Nat Rev Cancer 2015;15:701–11.
[3]
van Soest RJ, de Morree ES, Kweldam CF, et al. Targeting the androgen receptor confers in vivo cross-resistance between enza- lutamide and docetaxel, but not cabazitaxel, in castration-resistant prostate cancer. Eur Urol 2015;67:981–5.
[4]
Bovinder Ylitalo E, Thysell E, Jernberg E, et al. Subgroups of castra- tion-resistant prostate cancer bone metastases defined through an inverse relationship between androgen receptor activity and im- mune response. Eur Urol 2017;71:776–87.[5]
Hegde PS, Karanikas V, Evers S. The where, the when, and the how of immune monitoring for cancer immunotherapies in the era of checkpoint inhibition. Clin Cancer Res 2016;22:1865–74.[6]
Snyder A, Makarov V, Merghoub T, et al. Genetic basis for clinical response to CTLA-4 blockade in melanoma. N Engl J Med 2014;371: 2189–99.
[7]
Debets R, Donnadieu E, Chouaib S, Coukos G. TCR-engineered T cells to treat tumors: seeing but not touching? Semin Immunol 2016;28: 10–21.[8]
Bald T, Landsberg J, Lopez-Ramos D, et al. Immune cell-poor mela- nomas benefit from PD-1 blockade after targeted type I IFN activa- tion. Cancer Discov 2014;4:674–87.
[9]
Fong L, Carroll P, Weinberg V, et al. Activated lymphocyte recruit- ment into the tumor microenvironment following preoperative sipuleucel-T for localized prostate cancer. J Natl Cancer Inst 2014;106:dju268.
[10]
Sheikh N, Cham J, Zhang L, et al. Clonotypic diversification of intratumoral T cells following sipuleucel-T treatment in prostate cancer subjects. Cancer Res 2016;76:3711–8.[11]
Tumeh PC, Harview CL, Yearley JH, et al. PD-1 blockade induces responses by inhibiting adaptive immune resistance. Nature 2014; 515:568–71.E U R O P E A N U R O L O G Y 7 1 ( 2 0 1 7 ) 7 8 8 – 7 8 9
789